By subscribing, you agree to our Terms of Use and Policies You may unsubscribe at any time.
In an era where the effects of climate change are becoming increasingly evident, the need for renewable energy sources has never been more pressing. Solar energy stands at the forefront of this green revolution, offering a pathway to net zero. China Solar Panel
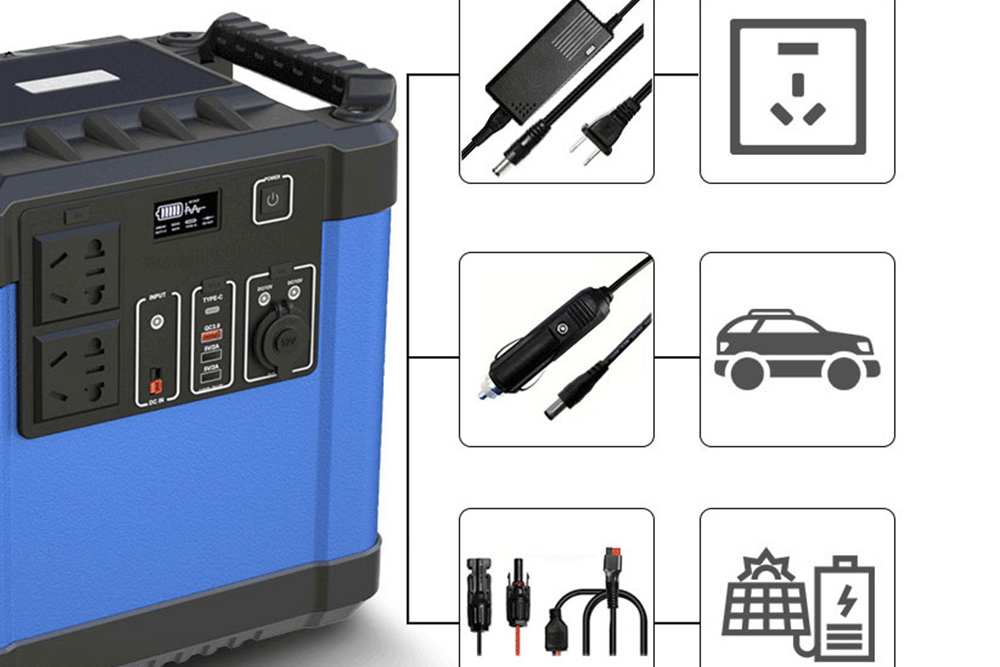
The Sun is, after all, our primary source of energy. On average, approximately 342 Watts of solar energy falls on every square meter of our planet's surface every year. This accumulation totals an astonishing 44 quadrillion (4.4 x 1016) Watts of power, a magnitude that would eclipse the output of 44 million sizable electric power plants.
Given the abundance of solar energy, it is surprising that it is not more widely harnessed and used. What is stopping its widespread adaptation?
Welcome to the ultimate guide to solar energy, where we embark on a journey to explore the power, potential, and promise of solar energy. We will explore everything from how solar panels work and their installation to recent advances in solar technology and the main challenges that come with solar energy.
Hopefully, this guide can answer all of your questions about solar energy and give you a new understanding and appreciation of solar technology.
At its core, solar energy is electromagnetic radiation emitted by the Sun. This radiant energy can be harnessed through various technologies to produce electricity, heat, and other forms of energy. Notably, solar energy is repleneshable (at least for the next few billion years or so) and significantly reduces environmental impact compared to fossil fuels.
The total amount of solar energy incident on Earth is thought to be more than adequate to meet the world's present and future energy needs.
The use of solar energy actually dates back to ancient civilizations, where passive solar techniques aided heating and lighting. For example, the early Greeks and Romans used magnifying glass and mirrors to light fires using the Sun's rays.
Another early use of solar energy was in Roman bathhouses. There, sunrooms used large windows to direct sunlight into a concentrated space.
Modern solar power could be said to have begun with our understanding of the photovoltaic (PV) effect, thanks to work by French scientist Edmond Becquerel. He determined that light could increase electricity generation when two metal electrodes were placed into a conducting solution.
The first selenium solar cell was invented by Charles Fritts in 1883, when he used selenium on a thin layer of gold to create a solar cell. This cell worked, but it had an efficiency of less than 1%.
Fritt's solar cell was not the most practical due to its low efficiency and its use of expensive gold. The solar cells used today are much more efficient and are made of cheaper and more plentiful silicon.
The silicon PV cell was invented by Calvin Fuller, Gerald Pearson, and Daryl Chapin at Bell Labs in 1954, marking the beginning of practical solar technology.
Today, solar power stands alongside wind, hydro, and geothermal sources as a rapidly expanding source of renewable energy. Solar energy also offers its own advantages in versatility and scalability, making it adaptable to diverse contexts, from small-scale residential installations to massive solar farms.
Now that we've got a little background about solar energy technology, we can dive into the science behind how it works—starting with PV technology.
The photovoltaic effect is a phenomenon in which semiconductors, commonly made of silicon, convert incident light into electric current by absorbing photons and releasing electrons. In simple terms, sunlight is converted into electric current. The device that does this is known as a photovoltaic, or PV cell, more commonly called a solar cell.
Solar cells are composed of silicon semiconductors, formed into a p–n junction. This is a boundary or interface between two types of semiconductor materials, dubbed p-type and n-type, located inside a single semiconductor crystal. Photons from sunlight create electron-hole pairs at this junction, generating electrons.
US Energy Information Administration
These electrons flow through the external circuit, producing usable electricity. A solar cell array or solar panel converts sunlight to direct current (DC), which an inverter converts to alternating current (AC).
Commonly made from silicon, other types of solar cell include organic, dye-sensitized, perovskite, and quantum dot cells, which use transparent conducting films on the illuminated side to allow light entry and charge collection.
Each of the various types of PV or solar cells comes with its own characteristics.
Monocrystalline cells, made from a single silicon crystal structure, stand out for their high efficiency and uniform appearance. However, their production process can be energy-intensive, leading to a higher carbon footprint.
Polycrystalline cells, composed of multiple silicon crystals, offer a cost-effective alternative and can be applied in diverse scenarios. However, they generally have slightly lower efficiency compared to monocrystalline cells, due to the crystal arrangement.
Thin-film cells, utilizing thin layers of semiconductors, provide flexibility that allows them to be employed in unconventional installations. Nonetheless, their efficiency tends to be lower than crystalline silicon cells, and they may require a larger surface area to produce the equivalent energy output.
As we can see, the choice of solar cell type often involves a trade-off between efficiency, cost, and specific application requirements. For example, monocrystalline cells are often found in home solar panels, due to their high efficiency (>20%) and capacity. However, their production can be costly due to silicon wastage.
Solar cells can be further classified into first, second, and third generations.
First-generation cells, often referred to as conventional or wafer-based cells, are primarily made of crystalline silicon. This technology includes materials like polysilicon and monocrystalline silicon, which have been the foundation of commercially predominant PV systems.
Second-generation cells, known as thin-film solar cells, encompass technologies such as amorphous silicon, CdTe, and CIGS cells. These thin-film cells find applications in diverse scenarios, from utility-scale PV power stations to building integrated PVs.
The third generation of solar cells represents emerging PV technology, characterized by thin-film technologies using organic and inorganic substances. Although many of these technologies are still in the research or development phase, they hold the promise of achieving low-cost, high-efficiency solar cells.
Despite the ongoing advancements, as of 2023, the most widely used and efficient solar cells remain those made from thin wafers of silicon, which also happens to be the oldest solar cell technology.
Efficiency and factors affecting performance
Solar panel efficiency is an important metric in determining how effectively sunlight is converted into electricity. Greater efficiency means that the solar panel can generate more electricity from the same amount of sunlight.
Not all of the energy captured from sunlight can be converted, and certain factors influence the overall efficiency of a solar panel system.
The proportion of sunlight that a solar panel can convert into usable power is referred to as conversion efficiency. It's an essential performance specification for a PV system, as it measures the maximum amount of electricity a panel can generate under peak conditions.
Cell type: Monocrystalline cells generally have higher efficiency than polycrystalline and thin-film cells because they're made from pure silicon.
Temperature: The efficiency of solar panels decreases as the temperature rises. This means that their performance may be affected by hotter temperatures.
Shading: This is a bit of an obvious one. Shading on a solar panel, even partially, can significantly reduce its output. Shade-tolerant designs and technologies can mitigate this effect.
Tilt and orientation: The angle and direction of solar panels affect their efficiency. Proper alignment with the Sun optimizes energy generation.
For optimal efficiency, aligning solar panels at an angle close to the latitude of the location, usually between 30 and 45 degrees, maximizes sunlight exposure and energy output. However, research has shown that this value varies depending on location.
From achieving complete energy autonomy to seamlessly integrating with the utility grid, solar energy systems provide a spectrum of possibilities.
Before we delve into solar energy systems, let's shed some light on an integral player in this arena—the power grid. The grid forms the backbone of our modern energy distribution, connecting power generators, transmission lines, and distribution networks to supply electricity to homes, businesses, and industries.
However, many regions which receive a lot of sunlight also lack access to a grid, making off-grid solar solutions attractive.
Off-grid solar systems provide a liberating approach to energy consumption. These setups are meant for those who seek autonomy from the grid, or those without access to a grid, and allow them to generate and store their own electricity.
Off-grid systems are particularly ideal for remote areas. They generally consist of solar panels, battery storage, charge controllers, and inverters.
Solar panels harness sunlight, converting it into electricity, which is then stored using batteries to ensure a constant power supply, even during periods of reduced solar energy production. This setup provides uninterrupted energy availability, regardless of fluctuating sunlight levels.
Efficient battery management is vital for off-grid solar systems. Batteries act as the primary energy store, which is crucial for stable power during cloudy days or high demand.
On-grid systems are designed to work with the existing power grid. An on-grid system consists of solar panels, inverters, and the grid connection itself. Solar panels capture sunlight and convert it into electricity, which is then channeled into the grid-connected inverter. The inverter transforms the DC produced by the panels into AC, aligning it with the grid's electrical characteristics.
The distinctive feature of on-grid systems is, of course, their connectivity to the power grid. Electricity generated by the solar panels is fed into the grid, effectively turning the electricity meter backward in a process known as net metering.
This allows you to accumulate credits for the energy you contribute, reducing utility bills.
When solar production is insufficient, such as at night or on cloudy days, the grid seamlessly provides the additional power required to run your home. This dual sourcing ensures a constant supply of electricity.
On-grid systems offer environmental benefits by reducing reliance on fossil fuels, and can significantly lower costs for users. They also help to balance grid demand and supply, leading to greater overall efficiency in the system. Their integration with the grid promotes a more sustainable energy mix and contributes to a reduction in carbon emissions.
Hybrid solar systems allows the homeowner to choose whether to direct the energy they generate to the grid or into a home battery system
A hybrid system consists of solar panels, battery storage, charge controllers, inverters, and grid connections, which work together seamlessly. The solar panels convert sunlight into electricity, which can power a home's immediate needs, charge the batteries for later use, and feed excess energy back into the grid.
The main advantage of hybrid systems lies in their ability to store surplus energy for later consumption. This is particularly beneficial during times of optimal solar production when energy generation exceeds usage.
Instead of returning excess electricity to the grid for net metering, it's stored in batteries for use during periods of low solar production or high energy demand.
Hybrid systems also offer a fail-safe mechanism. In the event that the batteries lose power and solar energy is insufficient, the system can seamlessly transition to receiving electricity from the grid. This flexibility is particularly helpful during unexpected power outages or extended periods of low sunlight.
Ultimately, the choice of solar energy systems depends on your specific needs and circumstances. Factors like energy goals, location, budget, and consumption patterns play a crucial role.
Whether you're seeking autonomy with an off-grid setup, savings with an on-grid system, or versatility with a hybrid solution, careful consideration and professional guidance can help you make an informed decision aligned with sustainability goals.
Solar energy systems are environmentally friendly and have several advantages that contribute to sustainable development. These systems exhibit lower greenhouse gas (GHG) emissions and considerably reduced pollution release during their operational lifecycle.
According to a study led by Atse Louwen from Utrecht University, the growth of solar energy has led to some clear benefits in sustainability. The study reveals that from 1975 onward there have been clear trends in cost reduction, cumulative energy demand (CED), and GHG emissions, all concurrent with the rapid growth of installed PV capacity.
Their findings indicate that, each time the capacity of PV systems doubles, the cost drops by approximately 20%. Alongside this cost reduction, there are positive changes in other areas, too.
The cumulative energy required decreases by between 11.9% and 12.6%, and greenhouse gas (GHG) emissions also decrease by approximately 16.5% to 23.6% with each doubling of capacity.
The growth of the PV industry initially acted as a net primary energy sink and emitted excess GHG emissions. However, the study's findings suggest that the environmental debt created during this growth phase was likely repaid by around 2011 for both CED and GHG emissions.
Even in the worst-case scenario, where environmental impacts are less favorable, the study shows a break-even point was reached in around 2017 for CED and 2018 for GHG emissions. From this point onward, any growth in solar energy led to a reduction in CED and GHG emissions.
Transitioning to solar energy offers a substantial opportunity to curtail carbon footprint.
According to a study led by Arshian Sharif, from Universiti Utara Malaysia, solar energy is an effective means of reducing the carbon footprint. Their findings align with previous studies suggesting that the carbon footprint lifecycle of solar energy is notably lower compared to coal or gas.
One illustration of this effect can be seen in domestic solar water heating systems. These systems emit 80% fewer GHG compared to their conventional counterparts, as seen in a study led by Muhammad Badar Hayat, from the Missouri University of Science and Technology,
The researchers also note that solar space heating systems present a 40% decrease in emissions compared to the use of conventional electric power plants.
While solar panels operate without water consumption, specific solar energy technologies, such as central tower systems, use water for cooling. This becomes a concern in arid regions which are often suited to solar energy generation but where water resources are scarce.
The increased water demand from such systems could exacerbate resource scarcity and even pose the risk of contaminating groundwater.
Although exact data on water use is available, this potential concern highlights the importance of carefully assessing the feasibility and environmental impact of solar energy installations in water-scarce areas.
Lifecycle analysis of solar panels
Although the production of solar panels involves the use of certain toxic and flammable materials, their environmental benefits far outweigh these initial impacts throughout their operational life.
For instance, it is estimated that the carbon footprint from the manufacture of a typical solar panel can be offset by its energy production in just three years. This signifies that the long-term positive impact of solar panels on greenhouse gas emissions significantly surpasses their initial environmental costs.
On average, the components of solar energy systems have a lifespan of around 25 to 30 years. But when we look at their environmental effects, we see that different components have different impacts.
Some components release pollutants like air, soil, and water contaminants more than others. This highlights the need to thoroughly evaluate each component to understand how it contributes to the overall environmental impact of the systems.
Sustainable practices in solar panel manufacturing
Recycling solar panels is essential for improving sustainability and recovering valuable materials. Various strategies have been explored, such as dismantling panels, using chemical and mechanical separation methods, and recovering precious metals like silver, tellurium, and indium.
While the full-scale implementation of these practices may still face challenges due to factors like collection logistics and economic viability, research and efforts are underway to maximize the recovery of valuable resources from used solar panels.
Incentives, policies, and financing are an essential part of the drive toward its widespread integration. As the world seeks cheaper, cleaner, and more sustainable energy solutions, these mechanisms will play a pivotal role in accelerating the transition to solar power.
One significant effort is the Solar Futures Study, a collaborative effort by the US Department of Energy Solar Energy Technologies Office (SETO) and the National Renewable Energy Laboratory (NREL).
Released in September 2021, this study forecasts the pivotal role of solar energy in achieving a carbon-free electric grid in the US. By leveraging aggressive cost reductions, government support, and large-scale electrification, the study suggests that solar could contribute as much as 40% of the nation's electricity supply by 2035.
Crucially, the study envisions achieving 95% decarbonization of the electric grid by 2035 without causing any increase in electricity prices.
In fact, the use of better technology and improved flexibility in energy demand is predicted to balance out the costs associated with reducing carbon emissions and transitioning to electrification.
Renewable Energy Certificates (RECs), also known as Renewable Energy Credits, are tradable certificates representing the environmental and renewable attributes of a certain amount of electricity generated from renewable sources.
When renewable energy is generated, it produces both electricity and environmental benefits, such as reduced GHG emissions. RECs provide a way to track and verify the environmental benefits of renewable energy production separately from physical electricity.
In line with that, Solar Renewable Energy Certificates (SRECs) highlight the environmental advantages of solar power and enhance grid reliability. This is evident in the comparison between solar panels and trees in offsetting carbon dioxide emissions.
Another effective government policy is illustrated by India's subsidy and incentive scheme for solar power projects. The government offers a subsidy of 12 Rupees (USD 0.14) per kilowatt hour for solar PV power and 10 Rupees (USD 0.12) per kilowatt hour for solar thermal power fed to the electricity grid.
Such schemes encourage investment by providing financial support to developers, thereby promoting the growth of solar energy infrastructure.
Community solar programs have also gained traction as way to make solar energy accessible to a broader number of people. These programs allow individuals and businesses to invest in shared solar projects, enabling those lacking rooftops or financial resources to benefit from the cost reduction of solar energy.
The growth of community solar is significant in the US, where it operates under a number of different models. SETO initiatives, like the National Community Solar Partnership and Solar in Your Community Challenge, also promote affordable solar access, especially for underserved communities.
Despite the promise of solar energy, financing remains a significant hurdle in realizing the wide-spread transition to renewables. Barriers to investment in clean energy production have included the affordability and availability of fossil fuels in certain regions, leading to fluctuating investment trends.
According to a study led by Sikandar Abdul Qadir, from Hamad Bin Khalifa University, Qatar, a significant challenge in clean energy investment comes from the affordability and availability of fossil fuels in some regions.
The study emphasizes the need for increased investment in clean resources like solar. The authors also highlight the importance of well-designed incentives and awareness campaigns to counter misconceptions about renewable energy costs.
The involvement of international corporations and tech giants, like oil companies and Amazon, can further influence the speed of renewable energy adoption.
The applications of solar energy span various sectors, showcasing its potential to transform multiple aspects of our lives.
As technology advances and incentives make solar more affordable, residential solar power is becoming an increasingly viable and beneficial option for homeowners looking to contribute to a sustainable future.
With multiple options, incentives, and financing plans available, transitioning to solar power has become more accessible. Beyond the compelling advantage of long-term cost savings, this is also playing a role in fostering a greener environment by reducing carbon emissions.
It's essential, however, to consider factors such as installation costs, the availability of sunlight, and the ongoing maintenance required to ensure optimal performance.
Agrivoltaic farming combines crop cultivation with solar panels, capitalizing on shared land use for food production and sustainable energy generation. Scientific studies have shown that certain crops can thrive when grown in this unique environment, opening up the potential to address global energy challenges and food security concerns.
This approach offers increased land-use efficiency by allowing solar farms and agricultural activities to coexist without competing for space. In this system, solar panels are generally elevated or suspended, providing space for crops. Solar panels placed on greenhouse roofs can also generate energy to power the greenhouses.
Notable examples of agrivoltaic farming include a project in South Korea where broccoli is grown underneath PV panels. Similarly, agrivoltaic systems in East Africa offer protection from heat stress and water loss for vegetables, enabling farmers to cultivate higher-value crops on previously challenging land.
With the global population projected to increase by 2 billion over the next three decades, agrivoltaic farming offers a means to produce both food and renewable energy on the same land, helping address the scarcity of available space.
Regions facing land constraints and seeking renewable energy solutions could benefit significantly from agrivoltaic farming.
Similar to residential solar power, industries can also significantly benefit from using solar energy to reduce their electric bills and contribute to environmental sustainability.
Installing solar power systems in factories and businesses makes it possible to cut electricity bills by up to 50% a month, resulting in substantial annual savings. Moreover, research shows these systems can also contribute to shading and cooling of buildings.
In some countries, accelerated depreciation policies allow industrial users to depreciate their solar power investments at a higher rate than fixed assets, resulting in tax savings and a more attractive return on investment.
Solar energy is driving a significant transformation within the transportation sector, particularly in regard to electric vehicles (EVs) and charging infrastructure. By incorporating solar panels directly into the design of charging stations, and even EVs themselves, sunlight could harnessed and converted into energy.
When used directly on EV roofs, solar panels extend the range and reduce reliance on external charging.
This innovation could also address a key concern among potential EV owners — range anxiety — and mitigate the fear of running out of battery power during longer journeys. Many solar charging stations already use panels to directly charge EVs or store energy for resilience during outages, resulting in lower carbon emissions associated with EV charging.
The integration of solar energy into transportation could also alleviate the load on the electrical grid, particularly during peak charging periods. By utilizing solar-powered EV charging, the energy demand is distributed more efficiently, leading to a more stable and efficient energy distribution system.
Beyond this, solar panels can also be integrated into public transportation systems such as buses and trains and, in fact, many cities around the world already have solar energy-powered public transportation.
EVs have been one of the early adopters of solar technology, and as this technology progresses, we can expect even more integration of solar panels into all types of vehicles.
Innovative applications of solar power extend to a variety of gadgets designed for use in daily life. This includes devices such as solar cookers that operate without direct sunlight and glowing jackets designed to enhance safety by attracting attention in the dark.
Other innovations include caps with built-in solar panels and a USB port; solar-powered outdoor lights in various shapes and sizes; and solar blinds for windows that integrate energy generation with shade.
For digital nomads, a solar charging backpack keeps electronic devices powered on the move, while music lovers could benefit from solar-powered Bluetooth speakers.
These everyday gizmos demonstrate just some of the potential of solar energy.
There have been a lot of recent advances in solar technology, from perovskite solar cells to the use of nanomaterials.
Perovskite is a naturally occurring mineral that has generated a lot of buzz in the solar industry. Solar cells made from perovskites have an efficiency exceeding that of conventional silicon cells.
Frequently, perovskite solar cells feature a hybrid organic-inorganic layer based on lead or tin halide materials, which serves as the active layer for capturing light. They are inexpensive and easy to manufacture, but their stability and scalability are a challenge.
Some recent studies have developed perovskite cells capable of achieving an efficiency of more than 15%. And, in November 2022, scientists led by Hao Chen from the University of Toronto set a new efficiency record for all-perovskite tandem solar cells.
This type of tandem solar cell involves stacking multiple layers of perovskite materials to capture a wide range of light wavelengths and enhance energy conversion. They achieved an efficiency of 27.4%, surpassing previous records and marking a significant advancement in perovskite solar cell technology.
Architecture is also getting involved, with building-integrated photovoltaics (BIPV). This includes the integration of PV materials into building structures, replacing traditional building components like roofs, facades, and skylights.
In BIPV systems, solar panels serve not only as energy generators but also as structural elements. The advantage of BIPV lies in potential cost savings through the integration of solar as well as reduced expenses on traditional building materials and labor for the replaced components.
Compared to stand-alone PV systems, BIPV's appeal lies in preserving the aesthetic integrity of a structure. BIPV is often considered preferable over conventional rack-mounted solar panels, which can disrupt the architectural design and appearance of a building.
BIPV technology can be integrated into various types of products, including standard in-roof systems, semi-transparent systems, cladding systems, solar tiles and shingles, and flexible laminates.
However, there are drawbacks — thermal performance can be affected due to higher operating temperatures, and costs are currently a barrier to adoption.
Beyond perovskite and BIPV, there is ongoing research into other emerging solar technologies. Organic solar cells, for instance, offer flexibility and transparency, opening up unique applications. However, their efficiency is very low at around 10%.
Quantum dot solar cells, based on nanoscale semiconductor particles, are another area of exploration, with the potential to effectively harness multiple wavelengths of light.
However, quantum dot solar cells also face challenges. Ensuring stability and preventing degradation over time is a concern due to their susceptibility to environmental factors.
Additionally, the complexity of synthesizing high-quality quantum dots and integrating them into functional solar cells remains an ongoing issue. Currently, quantum dot solar cells have an efficiency of around 18%.
Efficient energy storage is an essential component of solar energy. Recent advances in battery technology, particularly lithium-ion batteries, have greatly enhanced storage capacity, as well as compactness, performance, and longevity.
Despite challenges in battery lifespan, recycling, and costs, lithium-ion batteries are still gaining ground, due to improvements in affordability and reliability, and despite higher initial costs, lithium-ion batteries are competitive in terms of energy storage costs.
Apart from batteries, other solutions for solar energy storage are emerging, such as thermal storage. This stores excess energy as heat for later conversion to electricity and is used by the majority of solar thermal power facilities. For example, the Cerro Dominador Solar Power Plant in Chile can store 17.5 hours of energy as heat.
Artificial Intelligence (AI) and the Internet of Things (IoT) are also revolutionizing how we manage and optimize solar energy systems.
AI plays a crucial role in optimizing site selection, cutting construction costs, preventing delays, streamlining grid integration, enhancing performance forecasting, and improving demand scheduling.
Researchers at MIT and Stanford have developed a machine learning system that accelerates the optimization of perovskite-based solar cell production by incorporating data from experiments and human observations, resulting in higher-efficiency cells. This system aids in swiftly fine-tuning manufacturing methods for efficient and cost-effective solar cells.
IoT, on the other hand, refers to the interconnected network of physical devices, vehicles, buildings, and other items that are embedded with sensors, software, and connectivity.
These devices can collect and exchange data, enabling them to interact and work together without human intervention. In the context of solar energy, IoT involves the integration of smart monitoring devices, turning solar panels into interconnected nodes for remote monitoring, fault detection, and optimized performance in PV arrays.
Solar tracking systems are designed to maximize energy capture by orienting solar panels to follow the sun's path throughout the day. These systems come in two primary types: single-axis and dual-axis trackers.
Single-axis trackers move east-west with the sun, while dual-axis trackers move in all directions, ensuring panels are optimized for sunlight. These systems boost energy production, especially for big solar projects.
At the nanoscale, materials behave differently, and this property is being harnessed to enhance solar cell performance. Studies have shown that the use of nanotechnology in PV can bring significant benefits, such as enhanced light absorption and conversion efficiency, increased stability, and improved thermal performance. Nanomaterials are also being used to improve power conversion efficiency (PCE) and durability, crucial for prolonged functionality in diverse environments.
Despite these advantages, challenges such as the stability and potential toxicity of nanoparticles remain. For example, some nanomaterials, like carbon nanotubes (CNTs), are expensive to produce, impacting overall cost-effectiveness.
Complex integration processes and long-term reliability issues must also be addressed. Integration of nanotechnology in solar systems presents exciting opportunities, yet successful implementation requires a delicate balance between benefits and challenges.
And that’s a wrap! A lot is happening in the world of solar technology and hopefully, this article gave you some insight into these changes.
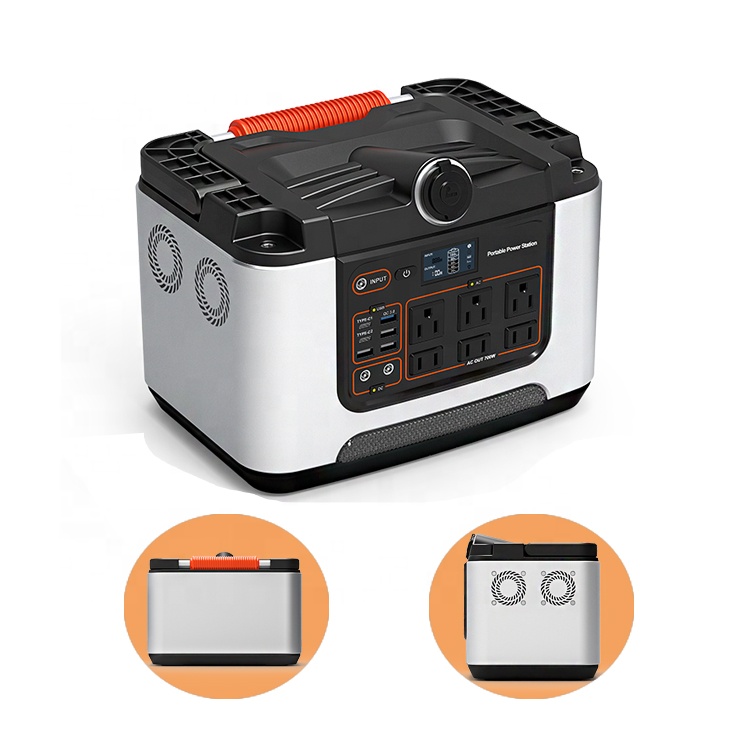
Portable Charger The sun's boundless energy, coupled with ongoing advancements in materials, engineering, and policy, is moving us toward a more sustainable future. As we navigate the challenges of climate change and energy security, embracing solar power is increasingly seen not just as an option, but as a necessity.